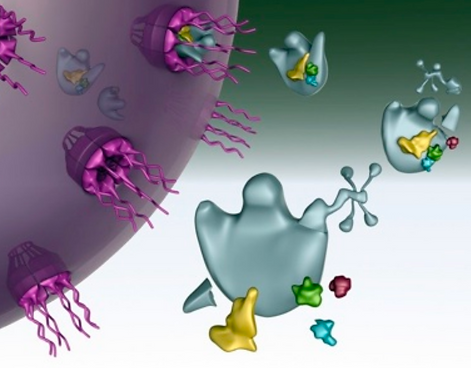
Nuclear export of ribosomes
​
Ribosomes are assembled in the nucleolus, a sub-compartment of the nucleus that serves as the cell's ribosome factory. However, they function in translation in the cytoplasm and therefore must be transported out of the nucleus. The selective transport of macromolecules into or out of the nucleus is controlled by the nuclear pore complex (NPC). So, the question is, how do ribosomes get through the nuclear pore complex. More than twenty years ago, we showed that the trans-acting factor Nmd3 shuttles in and out of the nucleus where it binds to nascent large (60S) ribosomal subunits to provide them with a nuclear export signal. The nuclear export signal of Nmd3 is a leucine-rich signal, a ligand for the export receptor Crm1. Because Crm1 has affinity for nucleoporins, the proteins of the NPC, it can usher the 60S subunit through the NPC. Crm1 is not the only receptor that the 60S subunit uses, but Nmd3 and Crm1 appear to be the only export factors conserved throughout eukaryotes. Yeast also use the mRNA export factors Mex67 and Mtr2 as well as the ribosome associated protein Arx1 for 60S export.
How do cells ensure that ribosomes are made correctly?
RNA modifications are widespread in biology, and particularly abundant in ribosomal RNA. However, the significance of these modifications is not well understood. We recently showed that methylation of a single universally conserved nucleotide, in the catalytic center of the large subunit, gates ribosome assembly. Massively parallel mutational scanning of the essential nuclear GTPase Nog2 identified important interactions with ribosomal RNA helix 92, particularly with the methylated A-site base Gm2922. We found that 2’-O-methylation of G2922 is needed for efficient nuclear export of the large subunit. We also identified single amino acid changes in Nog2 that completely bypass its dependence on G2922 methylation. By solving the cryo-EM structure of the unmodified nascent subunit, we revealed how methylation flips Gm2922 into the active site channel of Nog2. Our work showed how a single RNA modification is a critical checkpoint in ribosome biogenesis, and how RNA modifications can play an important role in regulation and assembly of macromolecular machines including the ribosome.
​
​
The cytoplasmic maturation of the large subunit
​
The nascent ribosomal subunits entering the cytoplasm are nearly fully assembled. However, they are not yet functionally active because they contain trans-acting factors that block their function and because they lack critical ribosomal proteins. Consequently, a number of remodeling events are required, driven by a host of ATPases and GTPases. Several years ago, we ordered the cytoplasmic maturation events of the 60S subunit into a hierarchical pathway. We showed that assembly of the P stalk is a prerequisite for the release of the subunit anti-association factor Tif6 as well as the export adapter Nmd3. During translation the stalk recruits and activates the GTPases that drive the ribosome through its translation cycle. The release of Tif6 depends Efl1, a paralog of the elongation factor eEF2. However, the release of Tif6 and Nmd3 also depend on assembly of the ribosomal protein Rpl10 into the subunit to complete the catalytic center. Defects in this step arrest biogenesis. Thus, we have proposed that Efl1 acts as a translation factor mimic to put the 60S subunit through a "test drive" to license the subunit for bona fide translation. There are a number of outstanding questions: Why do cells initially assemble inactive ribosomes? Is this a means of preventing the nascent ribosome from engaging in translation so that it can be transported to a desired location in the cytoplasm, akin to the transport of mRNAs in a translationally repressed state? What is the molecular basis for assess whether or not a newly made ribosome is functional? Are translation and ribosome biogenesis functionally coupled - that is, does the process of engaging in translation complete the biogenesis pathway? To begin to answer some of these questions and develop a mechanistic understanding of ribosome assembly we have turned to cryo-electron microscopy. We have begun to reconstruct the intermediates of cytoplasmic maturation, revealing a tightly choreographed series of protein exchanges and domain rearrangements during the assembly of the catalytic center. These structures reveal how the export adapter Nmd3 promotes the loading Rpl10 to complete the catalytic center provide mechanistic understanding of the hierarchical maturation pathway.
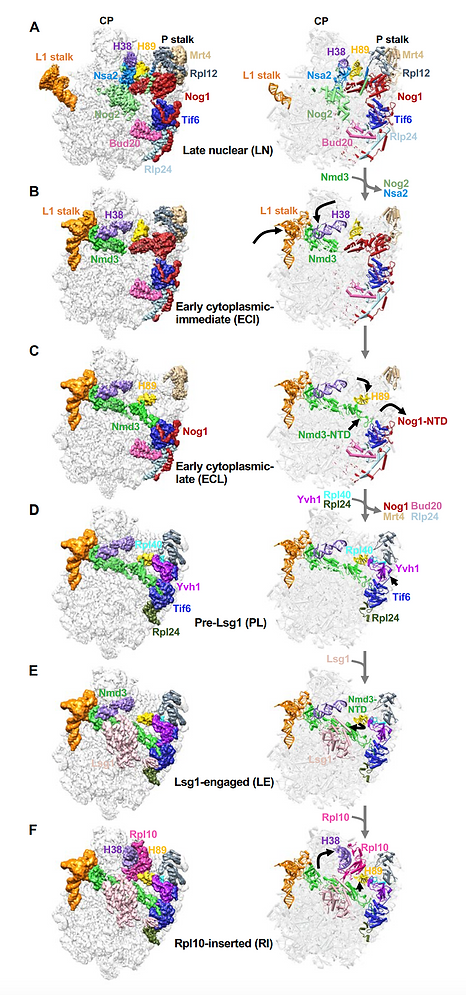

Forming the Central Pseudoknot of the small subunit
​
In eukaryotes, the highly conserved U3 snoRNA base-pairs to multiple sites in the preribosomal RNA (pre-rRNA) of the small subunit (SSU) to promote early cleavage and folding events. Binding of the U3 box A region to the pre-rRNA is mutually exclusive with folding of the central pseudoknot (CPK), a universally conserved rRNA structure of the small ribosomal subunit essential for SSU structure and protein synthesis. The U3 snoRNA is an essential component of a large complex called the SSU Processsome. Release of U3 is a critical step in the disassembly of the SSU Processome and the maturation of the SSU.
Our lab has made significant contributions in understanding the factors that drive the transition of the SSU Processome to the pre-40S. We have found that the DEAH-box helicase Dhr1 (Ecm16) is the enzyme responsible for displacing U3. Using a catalyically deficient Dhr1 mutants, we have been able to affinity purify a pre-40S particle containing U3 that remains base-paired with pre-18S rRNA. This particle contains a number of early-acting ribosome synthesis factors but noticeably lacks ribosomal proteins (r-proteins) that surround the CPK. The binding site of Dhr1 was mapped to U3 sequences flanking regions that base pair to the pre-rRNA including those that form the CPK. Point mutations in the box A region of U3 suppressed a cold-sensitive mutant of Dhr1, strongly indicating that U3 is an in vivo substrate of Dhr1. In collaboration with Dr. Carl Correll’s lab, we have shown that Dhr1 can unwind model U3 pre-rRNA duplexes. Dhr1 unwinding requires ATP but not ATP hydrolysis, reminiscent of the unwinding of substrates by DEAD-box helicases. We have also provided evidence that indicates that Utp14 regulates the unwinding activity of Dhr1 in vivo and in vitro. Moreover, Utp14 binds to the 5'-end, the middle, and 3'-end of the pre-rRNA where it is uniquely poised to monitor the status of transcription.
We have genetically linked Dhr1 and Utp14 to the RNA methyltransferase Bud23 which modifies G1575 of 18S rRNA. We have proposed that Bud23 recognition of its substrate works in conjunction with Utp14 to control the timing of Dhr1 displacement of U3. This may ensure that U3 is released only at the appropriate time in the RNA folding and subunit assembly pathway. bud23∆ mutants are suppressed not only by mutations in DHR1 and UTP14 but also by mutations in several other SSU Processome components, suggesting that a bud23∆ mutant is a sort of “genetic fulcrum.” Using this genetic approach, we are especially equiped to explore the events surrounding the disassembly of the SSU Processome.
Research
